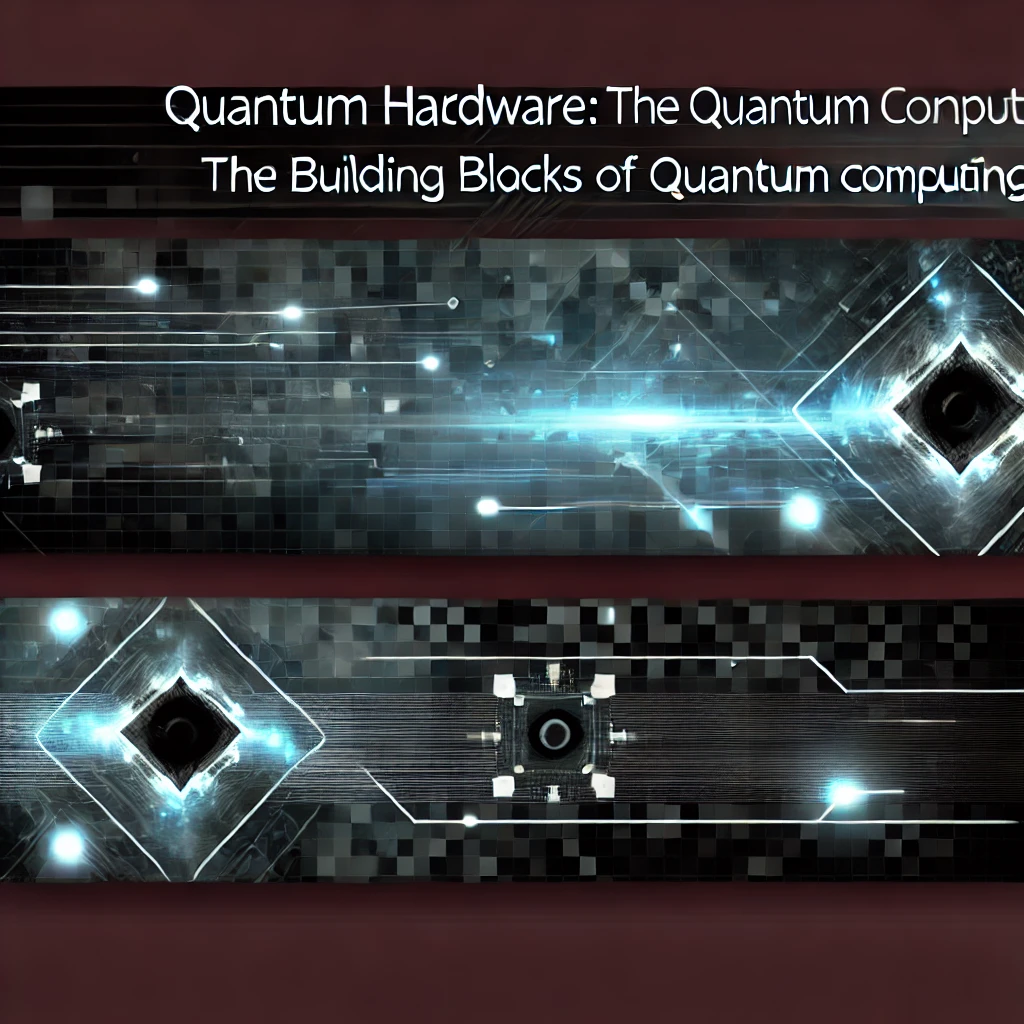
Quantum computing is often heralded as the next frontier in technology, promising to revolutionize industries ranging from cryptography to pharmaceuticals. At the core of this revolution lies quantum hardware—the physical systems that make quantum computation possible. In this blog, we will delve into the various types of quantum hardware, how they work, the challenges they face, and what the future holds for this exciting field.
What is Quantum Hardware?
Quantum hardware refers to the physical devices and systems that are used to build quantum computers. Unlike classical computers, which use silicon-based transistors to process information, quantum computers rely on quantum bits or qubits. These qubits exploit the principles of quantum mechanics, such as superposition and entanglement, to perform computations that are impossible for classical computers.
The Role of Qubits
Qubits are the fundamental units of quantum information. While classical bits can represent either a 0 or a 1, qubits can exist in a superposition of both states simultaneously. This property allows quantum computers to perform many calculations at once, exponentially increasing their computational power for certain tasks.
Types of Quantum Hardware
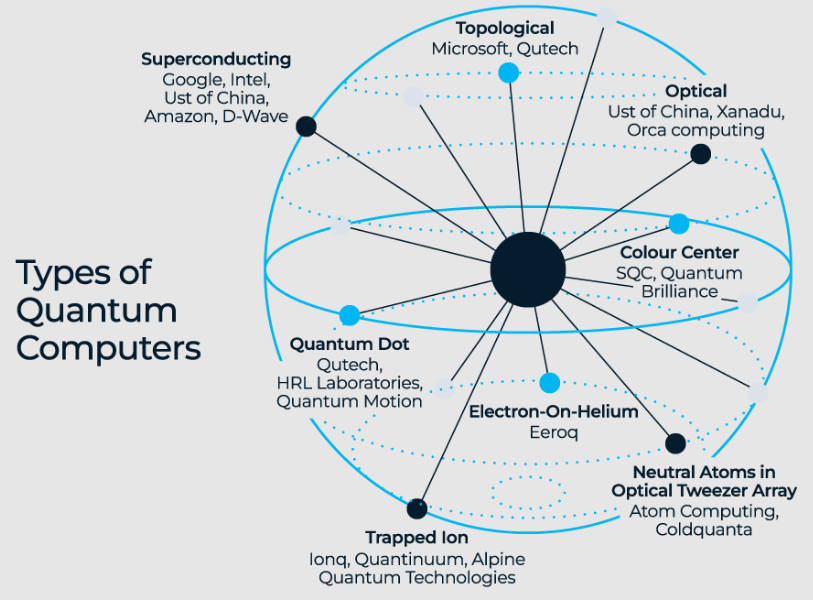
There are several different approaches to building quantum hardware, each with its own set of advantages and challenges. Below are some of the most prominent types:
1. Superconducting Qubits
Superconducting qubits are one of the most developed and widely used types of quantum hardware. These qubits are made from superconducting circuits that can carry current without resistance. They are typically implemented using Josephson junctions, which are devices that can control the flow of quantum information.
- How They Work: Superconducting qubits use microwave pulses to manipulate and measure quantum states. The qubits are cooled to near absolute zero to maintain their quantum coherence, which is essential for accurate computation.
- Advantages: Superconducting qubits are scalable and can be integrated into complex quantum circuits. They have been used in many of the most significant quantum computing experiments, including Google’s demonstration of quantum supremacy.
- Challenges: Superconducting qubits are highly sensitive to environmental noise, which can cause errors. Maintaining coherence over time is also a challenge, limiting the duration of computations.
2. Trapped Ion Qubits
Trapped ion qubits use individual ions (charged atoms) trapped in electromagnetic fields as qubits. These ions are manipulated using lasers to perform quantum operations.
- How They Work: The ions are confined in a vacuum chamber using electromagnetic fields. Laser pulses are used to control the quantum state of the ions, and entanglement between ions is achieved through their shared vibrational modes.
- Advantages: Trapped ion qubits have very high coherence times and can be manipulated with great precision. They are also well-suited for quantum error correction, a critical component of scalable quantum computing.
- Challenges: Scaling up trapped ion systems to include large numbers of qubits is challenging due to the complexity of controlling multiple ions simultaneously. The need for large, complex apparatuses also makes them less practical for integration into smaller, commercial devices.
3. Topological Qubits
Topological qubits are a more theoretical approach to quantum hardware, based on the principles of topological quantum computation. They use quasiparticles called anyons, which exist in two-dimensional spaces, to store and process quantum information.
- How They Work: Topological qubits rely on braiding anyons around each other to perform quantum operations. The quantum information is stored in the topological properties of the system, making it inherently protected from many types of errors.
- Advantages: Topological qubits are expected to be highly resistant to decoherence and noise, making them ideal for long-duration quantum computations.
- Challenges: Topological qubits are still largely theoretical, with significant challenges in creating and manipulating anyons in a controlled environment. Experimental realizations are in the very early stages.
4. Photonic Qubits
Photonic qubits use individual photons (particles of light) as qubits. These qubits are manipulated using optical devices like beam splitters, phase shifters, and interferometers.
- How They Work: Photonic qubits can be encoded in various properties of photons, such as polarization or phase. Quantum operations are performed by passing photons through optical circuits, and entanglement can be achieved using nonlinear optical processes.
- Advantages: Photonic qubits can be transmitted over long distances with minimal loss, making them ideal for quantum communication and distributed quantum computing. They also operate at room temperature, unlike other types of qubits that require extreme cooling.
- Challenges: Creating and detecting individual photons with high efficiency is challenging. Additionally, the interactions between photons are weak, making it difficult to perform certain types of quantum operations.
5. Silicon-Based Qubits
Silicon-based qubits are a newer approach that seeks to leverage existing semiconductor technology to build quantum computers. These qubits can be implemented using quantum dots or donor atoms in silicon.
- How They Work: Silicon qubits are typically created by isolating single electrons or nuclei within a silicon lattice. Quantum operations are performed using magnetic or electric fields.
- Advantages: Silicon-based qubits can potentially integrate with existing semiconductor manufacturing processes, making them scalable and commercially viable. They also benefit from the extensive knowledge and infrastructure developed for classical silicon-based electronics.
- Challenges: Maintaining coherence in silicon-based qubits is challenging due to interactions with the surrounding material. Additionally, scaling these systems while maintaining control over individual qubits remains a significant hurdle.
Challenges in Quantum Hardware Development
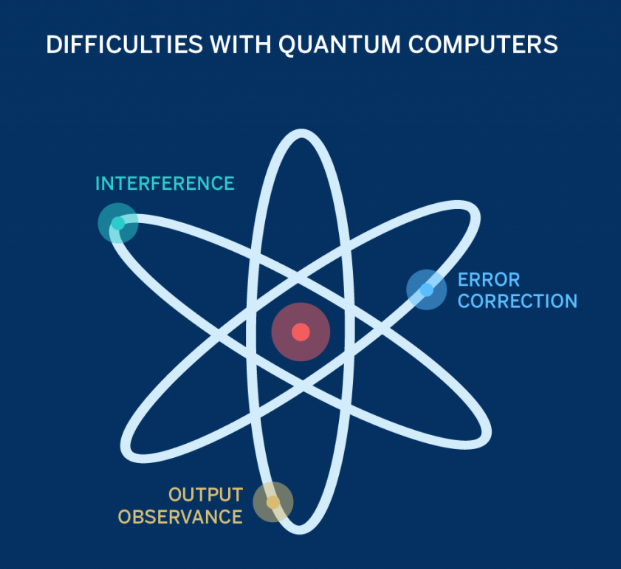
While quantum hardware has made significant strides in recent years, there are still many challenges that must be addressed before quantum computers can reach their full potential.
1. Error Rates and Quantum Error Correction
One of the biggest challenges in quantum hardware is the high error rates caused by decoherence and environmental noise. Quantum error correction is essential for building reliable quantum computers, but it requires a large number of qubits and complex algorithms to detect and correct errors without disturbing the quantum information.
2. Scalability
Scaling up quantum hardware to include millions of qubits is necessary for solving complex, real-world problems. However, maintaining coherence and controlling a large number of qubits simultaneously is a significant engineering challenge. Researchers are exploring various approaches to achieve scalability, including modular architectures and hybrid quantum-classical systems.
3. Cooling and Stability
Many types of quantum hardware, such as superconducting qubits and trapped ions, require extremely low temperatures to operate. Maintaining these conditions in a stable and energy-efficient manner is challenging, especially as quantum computers become larger and more complex.
4. Integration with Classical Systems
Quantum computers are not expected to replace classical computers but rather complement them. Integrating quantum hardware with classical processors and memory systems in a way that allows seamless communication and data transfer is another key challenge.
The Future of Quantum Hardware
The development of quantum hardware is advancing rapidly, with both academic institutions and private companies investing heavily in research and development. Here are some trends and future directions in the field:
1. Hybrid Quantum-Classical Systems
One promising approach is the development of hybrid systems that combine quantum and classical processors. These systems would use quantum computers to solve specific subproblems within larger computations, leveraging the strengths of both quantum and classical computing.
2. Quantum Cloud Computing
Quantum cloud computing is an emerging trend where quantum hardware is made accessible to users via the cloud. Companies like IBM, Google, and Microsoft already offer cloud-based quantum computing platforms, allowing researchers and developers to experiment with quantum algorithms without needing to own quantum hardware.
3. Advances in Quantum Materials
New materials with unique quantum properties are being explored for use in quantum hardware. These materials could enable more robust and scalable qubits, as well as new types of quantum devices that are currently theoretical.
4. Quantum Networking
Quantum networking involves connecting multiple quantum computers to create distributed quantum systems. This would enable quantum communication over long distances and allow for the creation of quantum internet infrastructure, which could revolutionize secure communication and distributed computing.
Conclusion
Quantum hardware is the foundation of the quantum computing revolution. While there are still many challenges to overcome, the progress made in recent years is paving the way for a future where quantum computers can tackle problems that are currently beyond the reach of classical systems. As research continues and new technologies emerge, quantum hardware will play a crucial role in shaping the future of computing.
Further Reading and Resources
For those interested in learning more about quantum hardware, here are some recommended resources:
- Books:
- “Quantum Computing: An Applied Approach” by Jack D. Hidary.
- “Quantum Computer Science: An Introduction” by N. David Mermin.
- Research Papers:
- Barends, R., et al. “Superconducting quantum circuits at the surface code threshold for fault tolerance.” Nature, vol. 508, no. 7497, 2014, pp. 500–503.
- Monroe, C., et al. “Large-scale modular quantum-computer architecture with atomic memory and photonic interconnects.” Physical Review A, vol. 89, no. 2, 2014, p. 022317.
- Online Courses:
- Coursera: Quantum Hardware for Beginners.
- edX: Quantum Mechanics for Scientists and Engineers.
FAQs
- What is the most widely used type of quantum hardware?
- Superconducting qubits are currently the most widely used type of quantum hardware, with companies like IBM and Google leading the development of superconducting quantum processors.
- Why are qubits so difficult to control?
- Qubits are highly sensitive to their environment, and even small disturbances can cause them to lose their quantum coherence. Maintaining precise control over qubits requires sophisticated technology and extreme conditions, such as ultra-low temperatures.
- How close are we to practical quantum computers?
- While significant progress has been made, practical quantum computers that can solve real-world problems are still likely years or even decades away. Current efforts are focused on improving qubit coherence, scaling up quantum systems, and developing practical quantum algorithms.
Thanks,