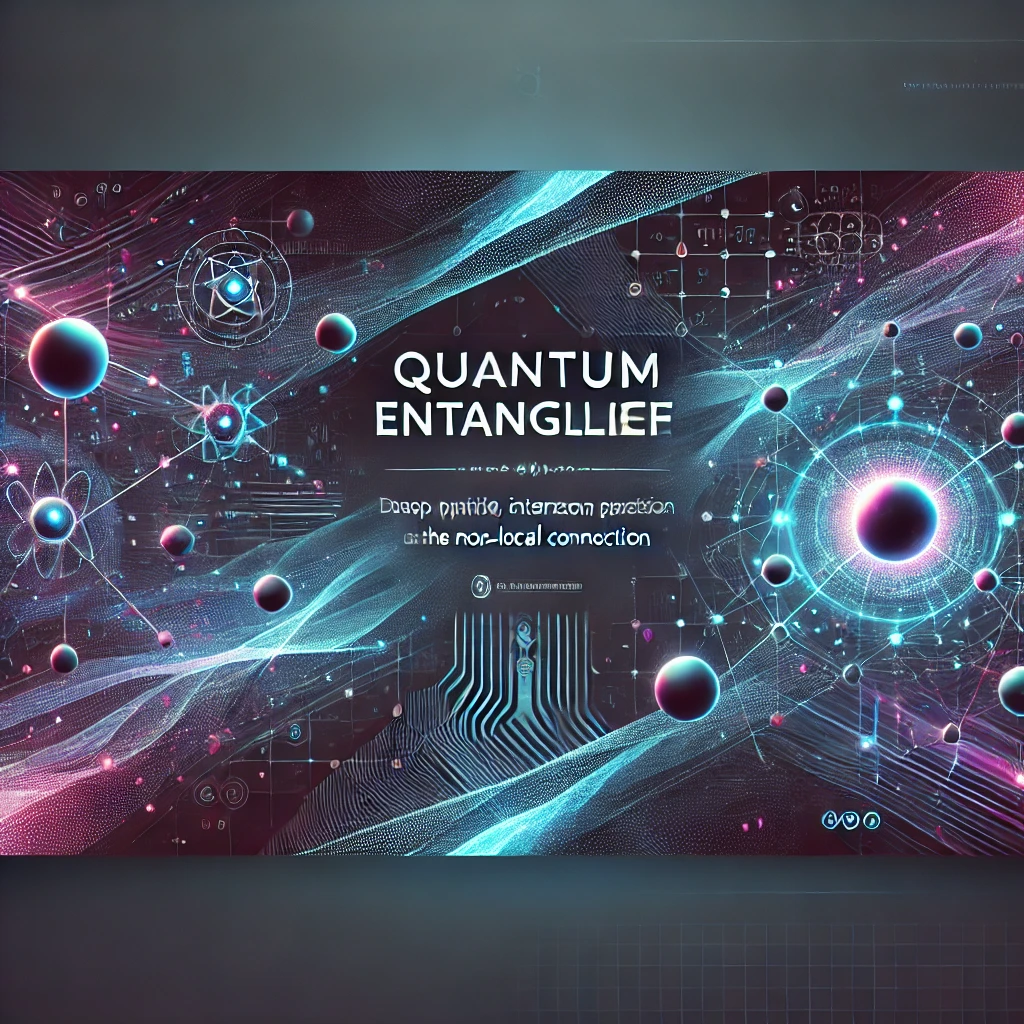
Quantum entanglement is one of the most intriguing and mind-bending phenomena in the world of quantum mechanics. It challenges our classical understanding of reality and has far-reaching implications for technology, communication, and even our philosophical outlook on the universe. In this blog, we will explore the depths of quantum entanglement, from its fundamental principles to its applications and future prospects.
What is Quantum Entanglement?
Quantum entanglement refers to a physical phenomenon that occurs when pairs or groups of particles become interconnected in such a way that the state of one particle cannot be described independently of the state of the other(s). This connection persists even when the particles are separated by vast distances, leading to what Albert Einstein famously called “spooky action at a distance.”
The Historical Roots of Entanglement
The concept of quantum entanglement was first introduced in the 1930s through the work of Albert Einstein, Boris Podolsky, and Nathan Rosen, in what became known as the EPR Paradox. They intended to challenge the completeness of quantum mechanics by demonstrating that it could lead to seemingly absurd conclusions, such as instant communication between distant particles. However, rather than disproving quantum mechanics, their work laid the groundwork for understanding one of its most profound mysteries.
Erwin Schrödinger, who coined the term “entanglement,” further developed the concept, recognizing its importance in quantum theory. Despite initial skepticism, entanglement has since been experimentally verified and is now a central feature of quantum mechanics.
The Physics of Quantum Entanglement
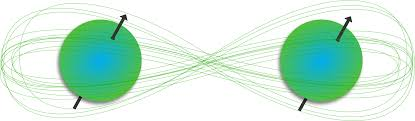
At the heart of quantum entanglement lies the principle of superposition, where particles exist in multiple states simultaneously until they are measured. When particles become entangled, their individual quantum states merge into a single, shared state. This means that the measurement of one particle instantly determines the state of its entangled partner, no matter how far apart they are.
Quantum States and Wave Functions
Quantum states are described mathematically by wave functions, which encapsulate all possible states of a system. For entangled particles, the wave function is not separable into individual components; instead, it represents a combined state. This non-separability is what makes entanglement so unique and difficult to reconcile with classical physics.
Bell’s Theorem and the Death of Hidden Variables
In the 1960s, physicist John Bell developed a theorem that provided a way to test whether the strange predictions of quantum mechanics—specifically, entanglement—could be explained by hidden variables, which would restore classical determinism. Bell’s theorem showed that no local hidden variable theory could reproduce all the predictions of quantum mechanics. Subsequent experiments, such as those by Alain Aspect in the 1980s, confirmed the non-local nature of quantum entanglement, effectively ruling out hidden variables and solidifying entanglement as a genuine physical phenomenon.
Quantum Entanglement and Non-Locality
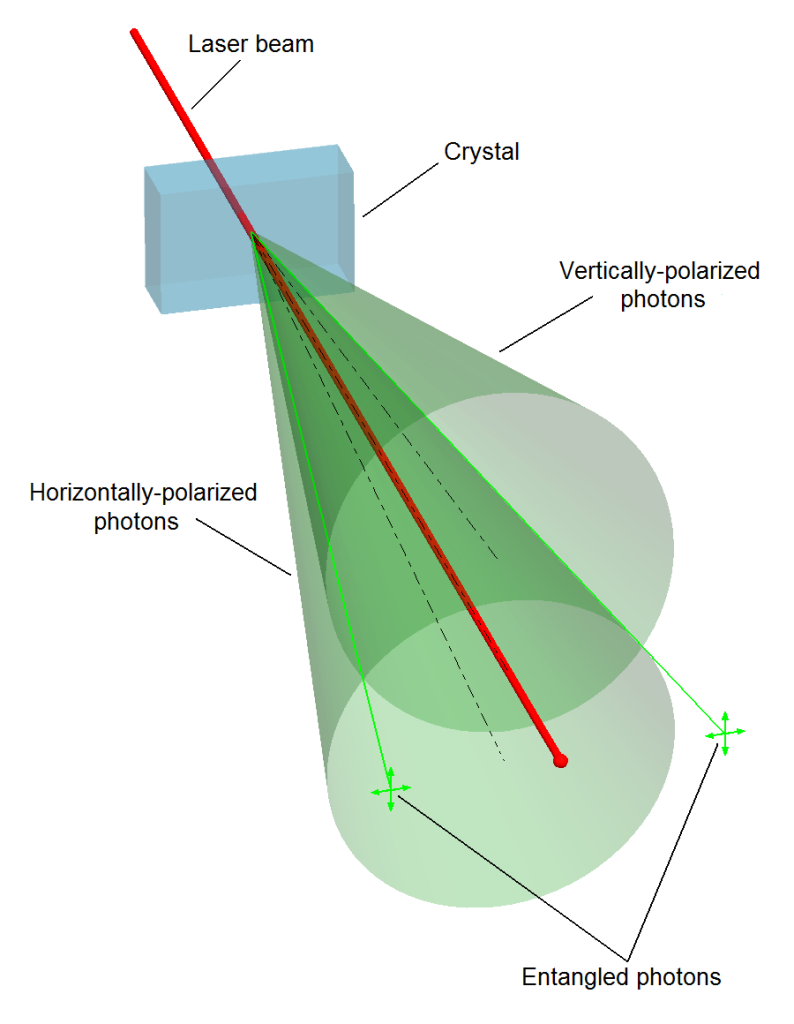
One of the most perplexing aspects of quantum entanglement is non-locality—the idea that entangled particles influence each other instantaneously, regardless of the distance between them. This defies the classical notion that information cannot travel faster than the speed of light.
Spooky Action at a Distance
Einstein’s discomfort with entanglement stemmed from this apparent violation of relativity, which forbids faster-than-light communication. However, it’s important to note that while entanglement involves instant correlations, it does not allow for the transmission of usable information faster than light, thus preserving the tenets of relativity.
Experiments Confirming Non-Locality
Numerous experiments have demonstrated the reality of non-locality. For instance, in 1982, Alain Aspect and his team conducted an experiment that measured the polarization of entangled photons. The results showed that changes in one photon’s polarization were reflected instantly in its entangled partner, even when separated by a significant distance.
Applications of Quantum Entanglement
Quantum entanglement is not just a theoretical curiosity; it has practical applications that could revolutionize technology and communication.
Quantum Computing
In quantum computing, entanglement is used to create qubits—quantum bits that can represent both 0 and 1 simultaneously, thanks to superposition. When qubits are entangled, they can perform complex calculations much faster than classical bits. This property is harnessed in algorithms like Shor’s algorithm for factoring large numbers, which has significant implications for cryptography.
Quantum Cryptography
Quantum entanglement also underpins quantum key distribution (QKD) protocols, such as BB84 and E91, which provide a secure method of communication. In QKD, any attempt to eavesdrop on the communication between two parties using entangled particles would disturb the system, alerting the parties to the intrusion and ensuring the security of the information.
Quantum Teleportation
Quantum teleportation is another fascinating application of entanglement. It allows the transfer of quantum information from one location to another without physically moving the particles themselves. This is achieved by entangling two particles, then using classical communication to transmit information about the state of one particle to the other, effectively “teleporting” the state.
Entanglement in Quantum Metrology
Entangled states are also used in quantum metrology, which involves making ultra-precise measurements. For example, entanglement can enhance the sensitivity of atomic clocks and improve the precision of interferometers used in gravitational wave detection.
Experimental Evidence and Challenges
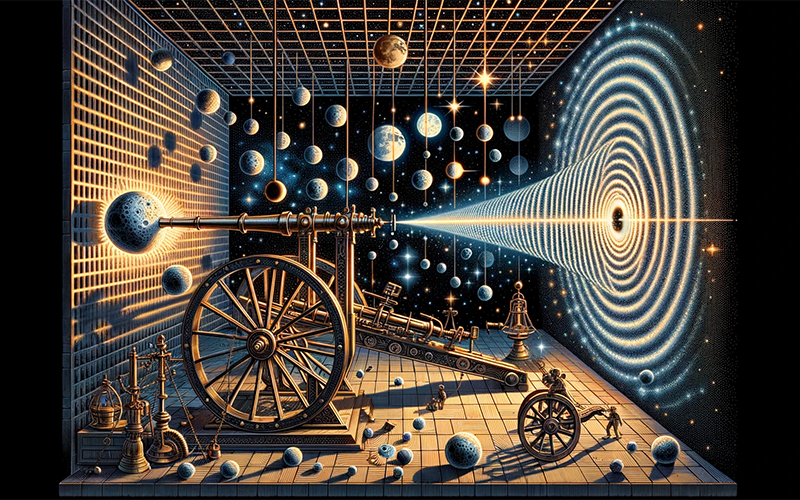
While quantum entanglement has been experimentally verified, there are challenges in maintaining and observing entangled states, particularly over long distances.
Notable Experiments
Experiments by John Clauser and Stuart Freedman in the 1970s, and later by Alain Aspect, provided early confirmation of quantum entanglement. More recently, experiments have successfully entangled particles over distances of several kilometers, paving the way for practical applications like quantum communication networks.
Technological Challenges
One of the main challenges in utilizing entanglement is decoherence, where interaction with the environment causes entangled states to lose their quantum coherence. Overcoming this requires advanced techniques to isolate and protect entangled particles, which is an area of ongoing research.
Theoretical Implications
Quantum entanglement challenges many classical concepts and has led to various interpretations of quantum mechanics.
Impact on Classical Concepts
Entanglement forces us to reconsider ideas like locality (the principle that objects are only directly influenced by their immediate surroundings) and realism (the belief that physical properties exist independently of observation). In the quantum world, entanglement suggests that these concepts may not hold.
Philosophical Interpretations
There are several interpretations of quantum mechanics that attempt to explain entanglement:
- Copenhagen Interpretation: This suggests that particles do not have definite properties until they are observed.
- Many-Worlds Interpretation: Proposes that all possible outcomes of quantum measurements actually occur in separate, branching universes.
- Bohmian Mechanics: Introduces hidden variables to restore determinism but requires non-locality.
Each interpretation has its strengths and weaknesses, and the debate over the “true” nature of quantum mechanics continues.
Quantum Field Theory and Entanglement
In quantum field theory, entanglement is not limited to particles but can also occur between different regions of space, leading to insights into the nature of space-time and potential connections to quantum gravity.
Philosophical and Ethical Considerations
The implications of quantum entanglement extend beyond physics into philosophy and ethics.
Nature of Reality
Quantum entanglement raises profound questions about the nature of reality. If particles can influence each other instantaneously across vast distances, what does that say about the interconnectedness of the universe? Some interpretations suggest that the universe is fundamentally holistic, with all parts intimately connected.
Ethical Implications
As quantum technologies develop, there are ethical considerations to address. For example, quantum cryptography could revolutionize security, but it could also be used for surveillance. The ability to manipulate and control quantum states also raises questions about the potential consequences of such power.
Future Prospects
Quantum entanglement is poised to play a central role in the future of technology and science.
Quantum Internet
One exciting prospect is the development of a quantum internet, where entangled particles are used to connect quantum computers over large distances. This could enable secure, high-speed communication and distributed quantum computing.
Advanced Quantum Algorithms
Researchers are exploring new quantum algorithms that leverage entanglement to solve complex problems in areas like drug discovery, materials science, and optimization.
Open Questions
Despite significant progress, many questions about quantum entanglement remain unanswered. For example, how does entanglement scale in complex systems? What role does entanglement play in black holes and the information paradox? These and other questions continue to drive research in the field.
Conclusion
Quantum entanglement is one of the most fascinating phenomena in modern physics, challenging our understanding of reality and offering potential for groundbreaking technologies. As research continues, we can expect to see even more exciting developments in this field, from quantum computers to secure communication networks and beyond.
Further Reading and Resources
For those interested in diving deeper into the world of quantum entanglement, here are some recommended resources:
- Books:
- Quantum Mechanics: The Theoretical Minimum by Leonard Susskind and Art Friedman.
- Entanglement: The Interplay of Quantum Mechanics and Information by Daniel Estève et al.
- Research Papers:
- Bell, J. S. “On the Einstein Podolsky Rosen Paradox.” Physics Physique Физика, vol. 1, no. 3, 1964, pp. 195–200.
- Aspect, Alain, et al. “Experimental Realization of Einstein-Podolsky-Rosen-Bohm Gedankenexperiment: A New Violation of Bell’s Inequalities.” Physical Review Letters, vol. 49, no. 2, 1982, pp. 91–94.
- Online Courses:
- MIT OpenCourseWare: Quantum Physics I, II, III.
- Coursera: Quantum Mechanics for Scientists and Engineers.
FAQs
- Can quantum entanglement be used for faster-than-light communication?
- No, while entanglement involves instant correlations, it does not allow for faster-than-light communication because it requires classical communication to interpret the results.
- How is quantum entanglement different from classical correlation?
- Classical correlation involves independent objects whose properties are correlated based on shared history or common cause. Quantum entanglement, however, means the properties of entangled particles are interdependent in a way that cannot be explained by any classical mechanism.
- What are some real-world applications of quantum entanglement today?
- Quantum entanglement is currently used in quantum key distribution for secure communications, and research is ongoing into its use in quantum computing and quantum teleportation.
Thanks,